Cell signaling, which is also often referred to as signal transduction or transmembrane signaling, is the process by which cells communicate with their environment and respond temporally to external cues that they sense there. The central importance of this general phenomenon (sensing of external stimuli by cells) has been appreciated for a long time, but it has truly become a dominant part of cell and molecular biology research in the past ten years. This approach lies at the core of the new field of proteomics, and its importance to human and animal health is already plainly evident. Cells within multi-cellular organisms communicate via extracellular mediators: either through diffusible molecules or by direct cell–cell contact. These extracellular signals are interpreted for the most part by specific membrane receptors that in turn trigger intracellular machinery that directs the cellular response. The past decade has seen an explosion in our understanding of the molecular basis for membrane receptor signaling.
Although cells from poly-cellular (multi-cellular) organisms derive substantial information from interactions with other cells and extracellular structural components, it was humoral components that first were appreciated to be intracellular messengers. This idea was certainly inherent in the “internal secretions” initially described by Claude Bernard in 1855 and thereafter, as it became understood that ductless glands, such as the spleen, thyroid, and adrenals, secreted material into the bloodstream. However, Bernard did not directly identify hormones as such. This was left to Bayliss and Starling and their description of secretin in 1902. Recognizing that it was likely representative of a larger group of chemical messengers, the term hormone was introduced by Starling in a Croonian Lecture, http://en.wikipedia.org/wiki/Croonian_Lecture, presented in 1905. The realization that these substances could also produce inhibitory effects, gave rise to a second designation, “chalones”, introduced by Schaefer in 1913 for the inhibitory elements of these glandular secretions. The word autocoid was similarly coined for the group as a whole (hormones and chalones). Although the designation chalone is occasionally applied to some growth factors with respect to certain of their activities (e.g., transforming growth factor β), autocoid has essentially disappeared. Thus, if the description of secretin and the introduction of the term hormone are taken to mark the beginnings of molecular endocrinology and the eventual development of cell signaling, then we are at or near the 100th anniversary of this field.
Ref:
Ralph A. Bradshaw and Edward A. Dennis, Handbook of Cell signaling Vol.1 (2003), 0-12-124547-0cAMP-dependent pathway:
When the ligand binds to GPCR, Gs type, the activated alpha subunit activates Adenylyl cyclase (effector) (EC 4.6.1.1) which converts ATP into cyclic AMP, 3'-5'-cyclic adenosine monophsophate,(as a second messenger).
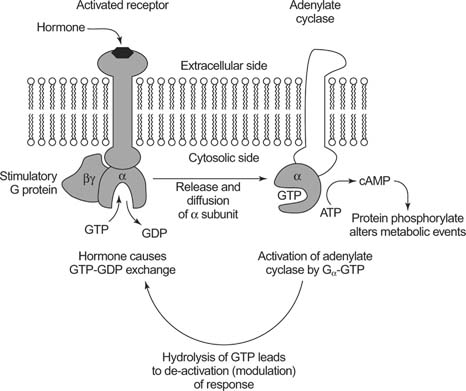

Action of cAMP:
A) Cyclic nucleotide-gated ion channel (CNG)
Cyclic nucleotides directly activate CNG channels by binding to a site on the channel protein. The dependence of channel activation on the ligand concentration is steep, indicating that several, most probably four, molecules of the ligand are required to fully open the channels. All CNG channels respond to some extent to both cAMP and cGMP. In rods and cones, CNG channels sharply discriminate between cAMP and cGMP, whereas channels in chemosensitive cilia of olfactory sensory neurons (OSNs) by and large respond equally well to both ligands. The ability to discriminate between ligands is commonly referred to as ligand selectivity. Selectivity can be achieved either by differential control of ligand affinity or efficacy or a combination of both. Ligand affinity is a measure of how tightly cyclic nucleotides bind to the channel. Efficacy refers to the ability to open the channel once the ligand has been seated in the binding cavity. Important functional features of these channels, i.e., ligand sensitivity and selectivity, ion permeation, and gating, are determined by the subunit composition of the respective channel complex. The function of CNG channels has been firmly established in retinal photoreceptors and in OSNs.
B) Exchange proteins activated by cAMP (Epac)
Rap guanine nucleotide exchange factor 3 (RAPGEF3) activated by cyclic AMP, which belongs to Guanine Nucleotide Exchange Factors, promotes the exchange of GTP for GDP bound to GTP-BINDING PROTEINS.
C) Protein Kinase A (PKA)
Phosphate groups are perfect chemical groups for modifying the function of proteins: they have a strong negative charge, they are fairly bulky, and they can form multiple hydrogen bonds. When a phosphate group is attached or removed to a protein, it may modify the shape and flexibility of the protein chain, or provide a readily-visible handle for recognition by other proteins. Cells take full advantage of these possibilities, and in a typical cell, phosphate groups are used to regulate the function of about one third of their proteins.
PKA is also known as cAMP-dependent protein kinase (EC 2.7.11.11). It is composed of two types of subunits. The catalytic subunit, shown here in pink, performs the phosphate-adding reaction. The regulatory subunit, shown in blue, senses the level of cyclic AMP, and turns the catalytic subunits on or off based on that level. When cAMP levels are low, a dimer of the regulatory subunits binds to two copies of the catalytic subunit, forming an inactive complex (shown at the top). When cAMP levels rise, it binds to the regulatory subunit, releasing the catalytic subunit in an active form (shown at the bottom).

PKA is an essential link in a cascade of messages that control energy utilization in cells. Receptors such as the beta-adrenergic receptor and the glucagon receptor stimulate the production of cyclic AMP. This activates PKA, which then phosphorylates many proteins involved in generation of cellular energy, regulating proteins like glycogen synthase and pyruvate kinase directly and also changing the synthesis of proteins by phosphorylating key transcription factors.
Specialized phosphodiesterases break the unusual cyclic phosphate bond in cAMP, forming AMP and making it inactive as a signaling molecule. Cells make many types of phosphodiesterases to tailor cAMP signaling for the needs of particular types of cells. Caffeine (the stimulant in coffee) and theophylline (the stimulant in tea) inhibit many forms of this enzyme, prolonging the energetic messages carried by cAMP. Drugs like Levitra and Viagra, on the other hand, inhibit only one type of phosphodiesterase.
The most important functions of PKA are phosphorylation of
- Enzymes that convert Glycogen into Glucose
- Enzymes that promote muscle contraction in the heart in order to increase heart rate
- Transcription factors (cAMP response element-binding protein, CREB).
*For detailed functions of PKA, kindly review the function table on http://en.wikipedia.org/wiki/Protein_kinase_A
Molecules that activate cAMP pathway include:
- Bucladesine (dibutyryl cAMP, db cAMP) - also a phosphodiesterase inhibitor [ It has vasodilator properties and is used as a cardiac stimulant]

1. http://www.sabiosciences.com/pathway.php?sn=cAMP_Pathway
2. http://www.cliffsnotes.com/study_guide/Cyclic-AMP-A-Second-Messenger.topicArticleId-24594,articleId-24544.html
3. http://physrev.physiology.org/content/82/3/769.long
4. http://www.ncbi.nlm.nih.gov/mesh/68020662
5. http://www.rcsb.org/pdb/101/motm.do?momID=152
6. http://en.wikipedia.org/wiki/CREB
7. https://www.medify.com/treatments/bucladesine
8. http://pubchem.ncbi.nlm.nih.gov/summary/summary.cgi?cid=9687
No comments:
Post a Comment